Improving fat oxidation at rest and during low-intensity exercise involves optimizing metabolic flexibility, mitochondrial efficiency, and hormonal balance.
Here are key strategies:
1. Nutritional Strategies
- Prioritize Fat Adaptation: Train your body to use fat more efficiently by incorporating a low-carb, high-fat (LCHF) or periodized carbohydrate approach. This doesn’t mean full keto but rather strategic carb intake based on training intensity.
- Time Carbohydrate Intake Wisely: Consume carbs around high-intensity workouts while keeping them lower at other times to enhance metabolic flexibility.
- Increase Healthy Fats: Include monounsaturated and polyunsaturated fats (avocados, olive oil, nuts, fatty fish) to support fat metabolism.
- Optimize Protein Intake: Adequate protein (not excessive) helps maintain muscle mass and metabolic rate without spiking insulin excessively.
2. Training Strategies
- Train in a Fasted State (Occasionally): Low-intensity steady-state (LISS) exercise in a fasted state (e.g., morning walks or easy rides) can enhance fat oxidation by depleting glycogen stores.
- Incorporate Zone 2 Training: Spending time in Zone 2 (60-70% of max heart rate) improves mitochondrial efficiency and fat oxidation capacity.
- Longer Aerobic Sessions: Extending the duration of low-intensity workouts (>60 minutes) helps shift energy utilization toward fat.
- High-Intensity Interval Training (HIIT): While HIIT primarily burns glycogen, it enhances mitochondrial biogenesis and metabolic flexibility, improving fat oxidation over time.
3. Lifestyle Factors
- Optimize Mitochondrial Health: Red light therapy, cold exposure, and sauna use can enhance mitochondrial function, supporting fat oxidation.
- Prioritize Sleep & Stress Management: Poor sleep and chronic stress increase cortisol, impairing fat oxidation and promoting carbohydrate dependency.
- Stay Hydrated & Optimize Electrolytes: Dehydration and imbalanced electrolytes can impair fat metabolism.
- Avoid Excessive Snacking: Allow time between meals to maintain stable insulin levels, promoting fat oxidation (metabolic flexibility).
4. Supplementation & Other Considerations
- L-Carnitine: Helps transport fatty acids into mitochondria for energy production.
- Exogenous Ketones or MCT Oil: Can support fat oxidation, especially during fasting or low-carb states.
- Caffeine: Enhances fat mobilization and oxidation during exercise.
- Metabolic Testing (PNOE, VO2 Max Testing): Helps assess your fat oxidation rates and personalize training/nutrition strategies.
Great point! Instead of using a percentage of VO₂ max, it’s more precise to determine Peak Fat Oxidation (FatMax) and structure training around it.
1. Determine Your FatMax Zone
- FatMax is the intensity at which fat oxidation is highest before carbohydrates become the dominant fuel source.
- This is typically found via metabolic testing (PNOE, VO₂ max test with substrate utilization data).
- FatMax is usually at the bottom of Zone 2, whereas the top of Zone 2 often sees a decline in fat oxidation as glycolysis takes over.
- If metabolic testing isn’t available, a rough estimate is around 60-65% of VO₂ max or ~70-80% of max HR, but this varies individually.
2. Optimize Fat Oxidation Training
- Train at or just below FatMax regularly: This means extended steady-state work (45-90+ minutes) at this intensity to build metabolic efficiency.
- Include fasted or low-glycogen sessions: Enhancing the body’s ability to use fat as fuel by training in a low-insulin state.
- Fuel strategically for higher intensities: Consume carbohydrates only when training at intensities above FatMax (i.e., higher Zone 2 and beyond).
3. Address the Zone 2 Deficiency Issue
- Many endurance athletes have a metabolic gap at the top of Zone 2, meaning their ability to oxidize fat drops off too soon, leading to early carbohydrate reliance.
- To improve this:
- Focus on low-intensity, high-volume work at the lower end of Zone 2.
- Perform periodic low-carb/fasted long sessions to enhance fat adaptation.
- Gradually push the top end of Zone 2 upward through progressive overload and mitochondrial efficiency training.
Mitochondrial Efficiency Training
Mitochondrial efficiency training enhances your ability to generate ATP with minimal byproducts (e.g., lactate, ROS) while maximizing fat oxidation. This is crucial for endurance performance and metabolic health.
Key Training Methods
1. Zone 2 Training (FatMax Focused)
- The foundation of mitochondrial efficiency is training at or slightly below your FatMax (bottom of Zone 2).
- This increases mitochondrial density, oxidative enzyme activity, and fat oxidation capacity over time.
- Sessions:
- 3-5x per week
- 60-120 minutes per session (depending on training load/recovery)
- Fasted or low-carb sessions to encourage fat adaptation (but not excessively to avoid under-recovery).
2. Long-Duration Low-Intensity Exercise
- Endurance sessions over 90 minutes at low intensity force mitochondria to become more efficient in utilizing fat for fuel.
- Helps improve fat oxidation rates and slow glycogen depletion during longer efforts.
- Example:
- 2-3 hour Zone 2 ride/run at FatMax effort.
3. High-Intensity Intervals (Mitochondrial Biogenesis)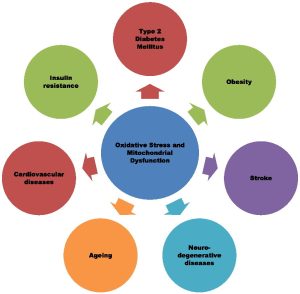
- While Zone 2 builds efficiency, short, high-intensity bursts trigger mitochondrial biogenesis through increased PGC-1α activation.
- Workouts:
- Sprint Intervals (e.g., 6-10 x 30s all-out efforts with 4 min recovery) improve mitochondrial quality.
- VO₂ Max Intervals (e.g., 4-6 x 3-5 min at 90-95% max HR with equal rest) push mitochondrial adaptation.
- Tabata-style (20s on, 10s off) helps increase mitochondrial enzyme activity.
4. Low-Glycogen Training (Selective Carb Restriction)
- Training with low glycogen forces mitochondria to rely more on fat and increases fat oxidation rates.
- Methods:
- Fasted morning Zone 2 workouts (but refuel afterward to support recovery).
- Second session of the day with low carb availability (e.g., first session depletes glycogen, second is done without refueling).
- Sleep low strategy (train in the evening, restrict carbs overnight, train low-intensity fasted in the morning).
Supporting Lifestyle & Nutrition
- Cold Thermogenesis: Increases mitochondrial density and efficiency (cold exposure 10-15 min post-training).
- Heat Adaptation: Sauna use post-workout promotes heat shock proteins and mitochondrial function.
- Nutritional Support:
- Creatine, L-carnitine, and PQQ improve mitochondrial function.
- Avoiding excessive processed foods that generate metabolic waste and inflammation.
Mitochondrial Efficiency Defined
Mitochondrial efficiency refers to how effectively mitochondria produce adenosine triphosphate (ATP), the body’s main energy currency, with minimal energy waste (e.g., heat, reactive oxygen species [ROS], and lactate). Efficient mitochondria maximize fat oxidation, improve endurance, and enhance overall metabolic health.
Key Aspects of Mitochondrial Efficiency
1. ATP Production Efficiency
- Mitochondria convert glucose and fat into ATP through oxidative phosphorylation.
- Higher efficiency means less energy is lost as heat and fewer metabolic byproducts are produced.
- Inefficient mitochondria rely more on glycolysis (carb burning) and generate excess lactate and ROS, leading to fatigue and inflammation.
2. Fat vs. Carbohydrate Utilization
- Well-functioning mitochondria preferentially burn fat at lower intensities and preserve glycogen for higher intensities.
- Poor mitochondrial function = early carb reliance, leading to bonking, insulin resistance, and metabolic inflexibility.
3. Mitochondrial Density & Biogenesis
- More mitochondria = greater energy production capacity and less metabolic stress per mitochondrion.
- Mitochondrial biogenesis (creation of new mitochondria) is triggered by exercise, fasting, cold exposure, and specific nutrients.
4. Oxidative Stress & Mitochondrial Dysfunction
- Inefficient mitochondria produce excessive ROS, leading to inflammation, aging, and chronic disease.
- Efficient mitochondria have strong antioxidant defenses (e.g., glutathione, SOD, catalase) to neutralize excess ROS.
Signs of Poor Mitochondrial Efficiency
- Fatigue & sluggish recovery
- Early carb dependency (poor fat oxidation)
- Poor endurance performance
- Brain fog & metabolic dysfunction
- Increased inflammation & oxidative stress markers
Mitochondrial Quality & Adaptation
Mitochondrial quality refers to the health, function, and efficiency of individual mitochondria, while mitochondrial adaptation refers to the process by which mitochondria improve in function, density, and efficiency in response to external stressors like exercise, fasting, and environmental changes.
1. Mitochondrial Quality: What Makes a Mitochondrion “Good”?
A. Functional Mitochondria vs. Dysfunctional Mitochondria
- High-quality mitochondria:
- Efficiently produce ATP with minimal energy loss.
- Preferentially oxidize fat at lower intensities and preserve glycogen.
- Generate fewer reactive oxygen species (ROS) and oxidative stress.
- Have a high ability to repair or recycle damaged components.
- Low-quality mitochondria:
- Struggle to produce ATP efficiently, leading to fatigue.
- Cause metabolic inflexibility (early reliance on carbohydrates).
- Generate excessive ROS, increasing inflammation and cellular damage.
B. Key Indicators of High Mitochondrial Quality
- High oxidative capacity (measured by metabolic testing, VO₂ max, or lactate threshold).
- Low ROS production (less oxidative stress and inflammation).
- Efficient fat oxidation (measured via respiratory exchange ratio [RER] or FatMax testing).
- Good mitochondrial turnover (efficient mitophagy—removal of damaged mitochondria).
2. Mitochondrial Adaptation: How to Improve Mitochondrial Quality
Mitochondria adapt to environmental and physiological stressors, improving their efficiency and function over time. Key adaptation mechanisms include:
A. Mitochondrial Biogenesis (Creating New Mitochondria)
- Controlled by PGC-1α (peroxisome proliferator-activated receptor gamma coactivator 1-alpha).
- Triggered by:
- Endurance training (Zone 2, FatMax training)
- High-intensity interval training (HIIT, sprint intervals)
- Cold exposure (activates AMPK and PGC-1α)
B. Mitochondrial Efficiency Upregulation
- Increased electron transport chain (ETC) efficiency = more ATP with less oxidative stress.
- Enhanced fat oxidation enzymes (CPT-1, beta-oxidation enzymes) = better metabolic flexibility.
- Strategies to improve:
- Low-intensity endurance training (FatMax work)
- Low-carb / fasted training sessions
- Nutrients like CoQ10, PQQ, creatine, and L-carnitine
C. Mitophagy (Removing Dysfunctional Mitochondria)
- Eliminates damaged, inefficient mitochondria that produce excess ROS.
- Triggered by:
- Fasting & caloric restriction (activates AMPK & autophagy).
- Heat exposure (sauna therapy).
- High-intensity exercise & hormetic stress.
D. Mitochondrial Fusion & Fission (Quality Control Mechanisms)
- Fusion: Combines damaged mitochondria with healthy ones for repair.
- Fission: Splits damaged mitochondria to remove dysfunctional parts.
- These processes maintain optimal mitochondrial networks and ensure efficient ATP production.
3. Practical Strategies to Enhance Mitochondrial Quality & Adaptation
A. Exercise-Based Strategies
- Zone 2 Training (FatMax Work) → Increases mitochondrial density & fat oxidation.
- HIIT / Sprint Intervals → Enhances mitochondrial biogenesis & efficiency.
- Low-Glycogen / Fasted Training → Forces mitochondria to rely on fat metabolism.
- Strength Training → Improves mitochondrial function in muscle fibers.
B. Lifestyle Strategies
- Cold Exposure (Cold Plunges, Cryotherapy) → Activates mitochondrial biogenesis.
- Heat Exposure (Sauna Therapy, Hot Baths) → Increases heat shock proteins & mitophagy.
- Fasting & Ketogenic States → Activates mitophagy and improves mitochondrial efficiency.
- Quality Sleep & Stress Reduction → Supports mitochondrial repair and recovery.
C. Nutritional & Supplementation Support
- CoQ10 & PQQ → Enhance mitochondrial function and energy production.
- L-Carnitine → Improves fat transport into mitochondria.
- Creatine → Supports ATP production and mitochondrial health.
- Polyphenols (Resveratrol, EGCG, Quercetin) → Boost mitochondrial biogenesis.
- Omega-3 Fatty Acids → Reduce mitochondrial inflammation.
Conclusion: Why Mitochondrial Quality & Adaptation Matter
- Better mitochondrial function = higher energy, improved endurance, faster recovery, and metabolic health.
- Training and lifestyle must be structured to encourage mitochondrial adaptation over time.
- Regular testing (PNOE, VO₂ max, lactate threshold, HRV) can track improvements in mitochondrial efficiency.
How Cold Exposure Activates Mitochondria
Cold exposure stimulates mitochondrial biogenesis, improves mitochondrial efficiency, and enhances fat oxidation through several key mechanisms.
1. Activates Brown Adipose Tissue (BAT) & UCP1
- Cold exposure (10–16°C / 50–60°F) stimulates brown fat activation, which contains high-density mitochondria.
- Brown fat mitochondria express UCP1 (uncoupling protein 1), which allows for non-shivering thermogenesis by generating heat instead of ATP.
- This increases mitochondrial activity and energy expenditure, improving metabolic efficiency.
Cold Exposure & Mitochondrial Biogenesis
Mitochondrial biogenesis refers to the process of creating new mitochondria, enhancing energy production, fat oxidation, and overall metabolic efficiency. Cold exposure is a potent stimulator of this process through several key mechanisms:
1. Activating PGC-1α (Master Regulator of Mitochondrial Biogenesis)
- Cold exposure stimulates PGC-1α, a key transcription factor that drives mitochondrial biogenesis.
- PGC-1α increases the production of proteins involved in oxidative phosphorylation (ATP production) and fat metabolism.
- This leads to an increase in mitochondrial density, particularly in brown fat and skeletal muscle.
2. Brown Adipose Tissue (BAT) & Mitochondrial Growth
- Cold exposure stimulates brown adipose tissue (BAT), which has a high mitochondrial density.
- BAT activation upregulates UCP1 (uncoupling protein 1), leading to increased mitochondrial activity and heat production.
- This process boosts mitochondrial biogenesis and enhances metabolic flexibility (fat burning instead of glucose).
3. AMPK Activation & Energy Stress Response
- Cold exposure induces AMPK (AMP-activated protein kinase), which signals low energy availability.
- AMPK activation triggers mitochondrial biogenesis and increases mitochondrial efficiency.
- This adaptation helps cells become more resilient to energy stress and improves endurance capacity.
4. Hormetic Stress & Reactive Oxygen Species (ROS) Signaling
- Brief cold exposure creates mild oxidative stress, which signals the body to adapt by producing more mitochondria.
- Controlled ROS production triggers Nrf2, which enhances antioxidant defenses and mitochondrial resilience.
- Over time, this adaptation leads to more efficient mitochondria that produce ATP with less oxidative damage.
5. Catecholamines (Norepinephrine & Epinephrine) & Mitochondrial Function
- Cold exposure increases norepinephrine release, which:
- Enhances fat oxidation and mitochondrial function.
- Stimulates thermogenesis and energy expenditure.
- Improves blood flow to mitochondria-rich tissues, increasing oxygen delivery.
Practical Application: How to Use Cold Exposure for Mitochondrial Biogenesis
- Cold Showers or Ice Baths (10-15 min at 50-60°F / 10-16°C, 2-4x per week).
- Cryotherapy Sessions (3-5 min at -110 to -150°C).
- Cold Water Immersion Post-Exercise (avoid immediately after strength training to prevent blunting hypertrophy).
- Outdoor Cold Exposure (exposing skin to cold air for short durations).
Benefits of Cold-Induced Mitochondrial Biogenesis
✔️ Increased fat oxidation & metabolic flexibility
✔️ Improved ATP production & endurance
✔️ Enhanced insulin sensitivity & glucose regulation
✔️ Reduced oxidative stress & inflammation
✔️ Greater resilience to environmental stressors
Custom Training & Lifestyle Plan for Mitochondrial Quality, Longevity, & Body Composition
This plan focuses on enhancing mitochondrial biogenesis, efficiency, and resilience through strategic exercise, nutrition, lifestyle habits, and targeted supplementation.
1. Training Plan: Improving Mitochondrial Density & Fat Oxidation
A. Aerobic Base (Zone 2 Training – 3-5x per week, 45-90 min sessions)
- Goal: Increase mitochondrial density, improve fat oxidation, and enhance metabolic flexibility.
- Intensity: 55-75% of max heart rate (below lactate threshold, at FatMax).
- Options:
- Fasted morning walks or low-intensity cycling.
- Steady-state swimming, rowing, or hiking.
- MAF training (Maximum Aerobic Function test to track improvements).
- Testing: Metabolic cart (PNOE, VO₂max) or lactate testing to find true FatMax zone rather than relying on % of VO₂max.
B. High-Intensity Interval Training (HIIT – 2x per week, 15-30 min sessions)
- Goal: Stimulate mitochondrial biogenesis & improve glucose uptake.
- Intensity: 85-100% of max effort (short bursts, long recovery).
- Protocol Options:
- Sprints: 4-8 rounds of 20-30 sec sprints, 2-3 min recovery.
- Rowing/Cycling: 30s max effort, 4 min recovery, 5-6 rounds.
- Stair sprints: 5 rounds of 30s all-out effort, 2-3 min rest.
- Tabata: 8 rounds of 20s work, 10s rest (for advanced athletes).
C. Strength Training (2-3x per week, Full-Body Focus)
- Goal: Increase muscle mass & metabolic health, which supports mitochondrial function.
- Type: Heavy resistance training with compound movements.
- Key Exercises:
- Deadlifts, squats, lunges (lower body mitochondrial demand).
- Pull-ups, push-ups, kettlebell swings (upper body & metabolic impact).
- Core work (planks, rotational exercises for stability).
- Reps & Sets: 3-5 sets of 5-12 reps, emphasizing time under tension.
D. Recovery & Regeneration (Essential for Mitochondrial Health)
- Daily mobility & stretching: Improves circulation and mitochondrial function.
- Breathwork (Nasal breathing, Box breathing, or Wim Hof method): Supports oxygen utilization in mitochondria.
- HRV Tracking: Use Oura ring, WHOOP, or HRV4Training to track recovery.
2. Lifestyle Strategies for Mitochondrial Adaptation
A. Cold Exposure (2-4x per week)
- Benefits: Stimulates mitochondrial biogenesis, enhances fat oxidation, and increases metabolic efficiency.
- Protocol:
- Ice baths (50-55°F, 2-5 minutes).
- Cold showers (30-60s exposure post-workout).
- Cryotherapy (if accessible).
B. Heat Exposure (3-5x per week, 15-30 min sessions)
- Benefits: Increases heat shock proteins (HSPs), mitophagy, and detoxification.
- Protocol:
- Sauna at 170-200°F for 15-30 min.
- Infrared sauna for deep tissue recovery.
- Hot baths for relaxation and circulation.
C. Fasting & Meal Timing (Mitochondrial Autophagy & Fat Adaptation)
- Intermittent Fasting (IF):
- 16:8 (fast for 16 hours, eat in an 8-hour window) to enhance mitophagy.
- Occasional 24-hour fasts (1-2x per month) to clear damaged mitochondria.
- Exercise-Fasted Workouts:
- Zone 2 sessions in a fasted state (optional).
D. Circadian Rhythm Optimization
- Morning Sun Exposure (10-15 min daily) to regulate mitochondrial function.
- Blue Light Blocking (2 hours before bed) for melatonin & mitochondrial repair.
- Consistent Sleep Schedule (7-9 hours per night) for optimal mitochondrial recovery.
3. Nutrition Plan: Fueling Mitochondrial Function
A. Macronutrient Strategy
- Protein: 1.6-2.2 g/kg body weight per day for muscle repair & mitochondrial support.
- Healthy Fats: Omega-3s, monounsaturated fats, and MCTs to support mitochondrial membranes.
- Smart Carbs: Cycle carbs based on training intensity (higher carb on strength/HIIT days, lower on rest days).
B. Mitochondrial-Boosting Foods
✔️ Fatty fish (Salmon, Sardines, Mackerel) – High in DHA, improves mitochondrial function.
✔️ Eggs – Choline & phospholipids support mitochondrial membranes.
✔️ Leafy Greens (Spinach, Kale, Swiss Chard) – High in magnesium & antioxidants.
✔️ Berries (Blueberries, Raspberries, Blackberries) – Polyphenols support mitochondrial health.
✔️ Dark Chocolate (85%+ cacao) – Contains flavonoids that enhance mitochondrial function.
✔️ Turmeric & Ginger – Anti-inflammatory, protect mitochondrial integrity.
C. Key Supplements for Mitochondrial Support
- CoQ10 / Ubiquinol – Essential for ATP production.
- PQQ (Pyrroloquinoline Quinone) – Stimulates mitochondrial biogenesis.
- Creatine Monohydrate – Supports ATP regeneration.
- L-Carnitine – Transports fatty acids into mitochondria for energy.
- Magnesium (Glycinate or Malate) – Required for ATP function.
- Alpha-Lipoic Acid (ALA) – Enhances mitochondrial antioxidant defense.
4. Longevity & Body Composition Optimization
A. Tracking Progress (Biofeedback & Testing)
- PNOE Metabolic Testing → Assess VO₂ max, FatMax, and mitochondrial efficiency.
- DEXA Scan or InBody Analysis → Track body composition changes.
- HRV Monitoring → Assess autonomic recovery & mitochondrial stress.
- Blood Work:
- Fasting insulin & glucose (HOMA-IR) → Assess metabolic flexibility.
- Lactate threshold & respiratory exchange ratio (RER) → Measure mitochondrial efficiency.
- Omega-3 Index Test → Ensure
How This Plan Differs for Middle-Aged Women vs. Men
While the foundational principles of mitochondrial health, longevity, and body composition optimization apply to both middle-aged women and men, hormonal differences require specific modifications, especially in perimenopausal and postmenopausal women.
Key Differences for Middle-Aged Women
- Hormonal Shifts & Recovery Needs
- Estrogen & progesterone decline impacts mitochondrial function, insulin sensitivity, and fat oxidation.
- Women may need more recovery time between HIIT or strength sessions due to a greater inflammatory response.
- Prioritize Zone 2 aerobic work & strength training over excessive HIIT, which can increase cortisol.
- Nutritional Adjustments
- Higher protein intake is essential (2.0-2.4 g/kg BW) due to anabolic resistance.
- Carbohydrate timing becomes more critical—women may need more carbs post-training to support insulin sensitivity.
- Omega-3s & phytoestrogens (flax, chia, soy in moderation) help support hormonal balance.
- Fasting & Metabolic Flexibility
- Women have a stronger HPA-axis response to fasting, so prolonged fasting (16:8+) may increase stress hormones.
- Instead, use a 12-14 hour overnight fast and avoid fasting on intense training days.
- Cold & Heat Exposure Considerations
- Women tend to have lower cold tolerance, so start with shorter cold exposure durations (1-3 min) and gradually increase.
- Heat exposure (sauna) is very beneficial for perimenopausal women to reduce hot flashes & enhance mitochondrial function.
- Tracking Recovery & Adaptation
- Women’s HRV tends to be more impacted by the menstrual cycle or perimenopause, so tracking HRV & sleep quality is key.
- Oura Ring, WHOOP, or HRV4Training can help monitor recovery needs.
Key Differences for Middle-Aged Men
- Testosterone Optimization
- Strength training & high-protein intake help preserve muscle mass & mitochondrial function.
- Men tend to tolerate fasting & HIIT better than women, but excessive endurance training can lower testosterone if not balanced.
- Fat Oxidation & Metabolic Health
- Men generally have greater fat oxidation capacity at rest and need less carbohydrate intake overall compared to women.
- Carb cycling based on training intensity still applies but with slightly lower post-workout carb needs than women.
- Cold Exposure Adaptation
- Men may tolerate longer cold plunges (up to 5 min at 50-55°F) due to higher BAT (brown adipose tissue) activation.
Functional Lab Tests for Personalized Program Design
To customize a mitochondrial health plan, these tests provide deeper insights into metabolic efficiency, hormonal balance, and recovery capacity:
1. Mitochondrial Function & Metabolic Efficiency
✅ PNOE Metabolic Test (VO₂max, FatMax, RER, Lactate Threshold) → Determines your true FatMax zone & metabolic efficiency.
✅ Organic Acids Test (OAT) → Identifies mitochondrial dysfunction, oxidative stress, and nutrient deficiencies (B vitamins, CoQ10, Carnitine).
✅ DUTCH Test (Dried Urine Test for Comprehensive Hormones) → Evaluates cortisol patterns, estrogen metabolism, & testosterone.
✅ Fasting Insulin & Glucose (HOMA-IR) → Measures insulin sensitivity & metabolic health.
2. Gut Health & Inflammation (Impacting Mitochondria & Energy)
✅ GI-MAP (Gut Microbiome Stool Test) → Assesses dysbiosis, inflammation, & gut infections affecting energy production.
✅ LPS (Lipopolysaccharides) & Zonulin Tests → Checks for leaky gut & mitochondrial stress from endotoxins.
✅ **hs-CRP & IL-6
Mechanism of Short-Chain Fatty Acids (SCFAs) & Butyrate in Mitochondrial Function, Fat Metabolism, and Inflammation
1. SCFA Production & Absorption:
- SCFAs (butyrate, acetate, and propionate) are metabolic byproducts of gut microbiota fermenting dietary fiber in the colon.
- Butyrate is the primary energy source for colonocytes and plays a direct role in mitochondrial function and systemic metabolic regulation.
2. Butyrate & Mitochondrial Function:
- Butyrate activates PGC-1α (Peroxisome Proliferator-Activated Receptor Gamma Coactivator 1-alpha), a master regulator of mitochondrial biogenesis and oxidative phosphorylation (OXPHOS).
- Enhances fat oxidation by increasing mitochondrial respiration and ATP production.
- Supports mitochondrial efficiency by reducing oxidative stress through increased antioxidant enzyme activity (SOD, catalase, glutathione peroxidase).
3. Butyrate & Fat Metabolism:
- Activates AMPK (AMP-activated protein kinase), promoting fatty acid oxidation and reducing lipogenesis.
- Upregulates PPAR-γ (Peroxisome Proliferator-Activated Receptor Gamma), enhancing lipid metabolism and insulin sensitivity.
4. Butyrate & Anti-Inflammatory Pathways:
- Inhibits NF-κB (Nuclear Factor Kappa B), reducing the production of pro-inflammatory cytokines (TNF-α, IL-6, IL-1β).
- Regulates T-regulatory (Treg) cells, promoting immune tolerance and reducing systemic inflammation.
- Strengthens gut barrier integrity by enhancing tight junction proteins (occludin, claudin, and ZO-1), preventing endotoxin leakage (LPS), which can impair mitochondrial function.
Why This Matters for Mitochondrial Health & Longevity:
- Increased butyrate levels = enhanced mitochondrial efficiency, improved fat metabolism, and reduced inflammation.
- Low butyrate production is linked to metabolic dysfunction, insulin resistance, and chronic inflammation—factors that accelerate aging and mitochondrial decline.
- Strategies like fiber-rich diets, resistant starch, and probiotic/prebiotic supplementation can optimize gut microbiome health and butyrate production.
Dr. Stacy Sims, a leading expert in female physiology, especially in relation to exercise, nutrition, and health optimization, provides recommendations specifically tailored for women’s unique hormonal and metabolic needs. Below are her key insights regarding mitochondrial health, training, and longevity optimization for middle-aged women:
Stacy Sims’ Recommendations for Mitochondrial Health and Longevity in Women
1. Hormonal Considerations
- Estrogen and Progesterone Decline (Perimenopause/ Menopause):
- As estrogen declines, women experience decreased muscle protein synthesis and increased fat storage, making it harder to maintain lean muscle mass and mitochondrial function.
- Dr. Sims emphasizes that post-menopausal women should focus on maintaining strength training and proper protein intake to counteract muscle loss and support metabolic health.
2. Exercise Protocol for Women
- Zone 2 Training (Aerobic Base):
- Dr. Sims recommends Zone 2 aerobic training (where you are still able to talk but feel like you’re working) as the foundation of a mitochondrial health program.
- For women, Zone 2 is essential for improving fat oxidation (using fat as the primary fuel source), which can support body composition and metabolic flexibility.
- Women may also benefit from shorter, more frequent workouts versus long, intense sessions, especially if they are experiencing hormonal fluctuations.
- Strength Training:
- Dr. Sims advocates for strength training 2-3 times a week, with an emphasis on compound movements (squats, deadlifts, lunges, push-ups, etc.), as these recruit the largest muscle groups and stimulate mitochondrial biogenesis.
- Progressive overload is key: gradually increasing weights helps build muscle mass and maintains mitochondrial density.
- HIIT (High-Intensity Interval Training):
- While HIIT can be effective, Dr. Sims advises modulating intensity and recovery time, as women’s hormonal fluctuations (especially during perimenopause and menopause) can lead to a greater stress response if HIIT is overdone.
- Women should aim for 2x per week of HIIT, focusing on quality (proper recovery and intensity balance) rather than sheer volume.
3. Nutrition for Mitochondrial Function and Longevity
- Protein Needs:
- Dr. Sims emphasizes that women in perimenopause or menopause need more protein than they did before (around 1.6–2.0 g/kg of body weight), as anabolic resistance increases with age.
- Include high-quality protein (whey, eggs, fish, lean meats) in each meal and snack, particularly around workouts (e.g., 15-20g of protein post-exercise). This helps with muscle repair and supports mitochondrial function.
- Carbohydrate Cycling:
- Carbohydrates should be timed around exercise: For low-intensity aerobic work, carbs should be kept low, but for higher-intensity exercise (e.g., strength training, HIIT), 30–40g of carbs pre-workout can support energy demands without overloading insulin.
- Cycle carbs based on training: Women should adjust their carbohydrate intake depending on their training volume and intensity, with more carbs on heavy training days (strength/HIIT) and lower carbs on rest days.
- Healthy Fats:
- Omega-3s (e.g., from fish oil or flaxseeds) are essential for mitochondrial membranes and reducing inflammation.
- Dr. Sims recommends incorporating monounsaturated fats (e.g., olive oil, avocado) and omega-3-rich foods (salmon, walnuts) to support mitochondrial membrane fluidity.
4. Recovery and Sleep
- Rest & Recovery:
- Recovery is essential, especially for women dealing with hormonal shifts. Dr. Sims advocates for active recovery days, incorporating mobility, yoga, and stretching.
- Adequate sleep (7-9 hours) is crucial for mitochondrial repair and optimal fat loss.
- Women should be mindful of sleep quality, ensuring they avoid blue light exposure at night and optimize their sleep environment (dark, cool, and quiet).
5. Cold & Heat Exposure
Cold Exposure:
- Dr. Sims highlights that cold exposure can be especially beneficial for women during perimenopause and menopause as it may help manage hot flashes, support fat oxidation, and enhance mitochondrial biogenesis.
- She recommends starting with short cold exposure (1-3 minutes) and gradually working up to cold showers or ice baths as tolerated.
- Heat Exposure:
- Sauna use can be highly beneficial for women as it helps with heat shock protein production, supports mitochondrial function, and may alleviate hot flashes.
- 15-30 minutes in a sauna (or infrared sauna) 2-3 times a week can help improve cardiovascular health, reduce stress, and support metabolic flexibility.
6. Supplementation for Mitochondrial Health
- Dr. Sims recommends key supplements to optimize mitochondrial function:
- CoQ10 (ubiquinol form for better absorption) – Supports ATP production.
- Magnesium – Helps with mitochondrial energy production.
- Alpha-lipoic acid (ALA) – Powerful antioxidant, aids in glucose metabolism.
- L-carnitine – Supports fat oxidation by helping fatty acids enter the mitochondria for energy.
- Vitamin D – Supports mitochondrial health and hormone balance.
Summary of Stacy Sims’ Approach for Middle-Aged Women
- Training: Focus on Zone 2 aerobic work, strength training (2-3x per week), and modulated HIIT (1-2x per week). Prioritize recovery and manage training volume to balance hormonal fluctuations.
- Nutrition: Increase protein intake, cycle carbohydrates based on training intensity, and include healthy fats to support mitochondrial function and longevity.
- Recovery: Prioritize sleep, active recovery, and cold/heat exposure to enhance mitochondrial biogenesis and stress resilience.
- Supplements: Consider CoQ10, magnesium, ALA, L-carnitine, and vitamin D to support mitochondrial efficiency and overall health.
Incorporating these principles will not only support mitochondrial health but also help women maintain optimal body composition, energy levels, and longevity as they age.
1. Mitochondrial Function & Metabolic Health
These tests assess mitochondrial function, oxidative stress, fat metabolism, and the ability to burn fat as an energy source.
- PNOE Metabolic Test
- Measures VO₂max, FatMax, lactate threshold, and respiratory exchange ratio (RER).
- Helps identify the optimal fat-burning zones and metabolic flexibility, which is crucial for mitochondrial efficiency and fat loss.
- Organic Acids Test (OAT)
- This comprehensive test measures markers that indicate mitochondrial dysfunction (e.g., citric acid, alpha-ketoglutarate), oxidative stress, and nutrient deficiencies (e.g., B vitamins, CoQ10, carnitine, acetyl-CoA).
- It also identifies toxins and gut metabolites that may affect mitochondrial health.
- Citrulline Malate
- A test that assesses amino acid profiles, including markers for mitochondrial energy production.
- Lactate & Pyruvate Test
- Measures lactate levels, indicating anaerobic threshold. Elevated lactate may suggest impaired mitochondrial efficiency or insufficient oxygen delivery to the muscles.
2. Hormonal Health and Aging
As mitochondrial function is closely tied to hormonal balance, particularly sex hormones in women, testing hormones can shed light on how to optimize both metabolic health and mitochondrial performance.
- DUTCH Test (Dried Urine Test for Comprehensive Hormones)
- A comprehensive test for sex hormones (estrogen, progesterone, testosterone), cortisol patterns, and melatonin.
- Useful for assessing how hormonal imbalances (especially during perimenopause/menopause) might be affecting mitochondrial function, fat metabolism, and overall energy.
- Thyroid Function (TSH, Free T3, Free T4, Reverse T3)
- Thyroid hormones play a pivotal role in mitochondrial efficiency and energy metabolism.
- Imbalances (like hypothyroidism) can impair fat loss and mitochondrial function, making it important to assess.
3. Inflammation & Oxidative Stress
Mitochondria are highly susceptible to oxidative stress, which can impair their function. Measuring inflammation and oxidative stress helps identify factors contributing to poor mitochondrial health.
- hs-CRP (High-Sensitivity C-Reactive Protein)
- A marker of systemic inflammation. Elevated levels can signal chronic inflammation, which can contribute to mitochondrial dysfunction and hinder fat loss and longevity.
- Oxidative Stress Panel (8-OHdG, F2-isoprostanes)
- Measures markers of oxidative damage at the DNA level. Elevated oxidative stress can damage mitochondrial membranes, impair energy production, and accelerate aging.
- Glutathione Levels (Total, Reduced, and Oxidized)
- Glutathione is a major antioxidant produced by the mitochondria. Low levels of reduced glutathione suggest mitochondrial dysfunction and may indicate a need for additional support.
4. Insulin Sensitivity & Fat Metabolism
Mitochondrial health is closely linked to how effectively the body processes glucose and fat for energy. These tests help assess metabolic flexibility.
- Fasting Insulin & Glucose (HOMA-IR)
- Insulin resistance (IR) negatively affects mitochondrial function and the ability to burn fat. This test measures insulin sensitivity and the HOMA-IR index (a ratio of fasting glucose to insulin levels).
- A1C (Hemoglobin A1C)
- This test reflects average blood glucose levels over the past 3 months. Elevated levels may indicate poor metabolic flexibility and an increased risk of diabetes or insulin resistance, which affect mitochondrial function.
- Fasting Lipids (Triglycerides, LDL, HDL)
- Abnormal lipid profiles (e.g., elevated triglycerides or low HDL) can signal insulin resistance and impaired fat metabolism, which impact mitochondrial health and fat loss.
5. Gut Health
Mitochondrial function is closely linked to gut health, as the gut microbiome can influence inflammation and nutrient absorption essential for mitochondrial health.
- GI-MAP (Gut Microbiome Stool Test)
- Analyzes the gut microbiome and assesses levels of beneficial bacteria, dysbiosis, pathogens, and markers for intestinal permeability (leaky gut).
- Imbalance in the gut microbiome can increase systemic inflammation, impair nutrient absorption, and impact mitochondrial health.
- Zonulin & LPS (Lipopolysaccharides)
- These tests measure gut permeability and the impact of endotoxins (from bacteria) on mitochondrial function. Leaky gut and elevated LPS are associated with mitochondrial stress and fat storage.
- Gut Health & Inflammation (Impacting Mitochondria & Energy):
- ✅ hs-CRP & IL-6 (High-Sensitivity C-Reactive Protein & Interleukin-6) → Markers of systemic inflammation. Chronic inflammation from poor gut health, endotoxins, or dysbiosis can impair mitochondrial function and energy metabolism.
- ✅ Calprotectin & Secretory IgA (sIgA) → Measures intestinal inflammation and immune response. Chronic gut inflammation can increase oxidative stress and impair mitochondrial efficiency.
- ✅ Short-Chain Fatty Acids (SCFAs) & Butyrate Levels → Assess gut microbiome diversity and butyrate production. Butyrate fuels mitochondrial function and helps regulate fat metabolism and anti-inflammatory pathways.
- ✅ Oxidative Stress Markers (8-OHdG, F2-isoprostanes) → Evaluate oxidative damage and mitochondrial dysfunction linked to gut-driven inflammation.
- These tests collectively provide insights into how gut health impacts mitochondrial efficiency, fat oxidation, and longevity by addressing dysbiosis, endotoxin exposure, and chronic inflammation that can impair mitochondrial ATP production.
6. Nutrient Deficiencies
Deficiencies in key micronutrients play a significant role in mitochondrial efficiency. These deficiencies can hinder fat loss, energy production, and longevity.
- Vitamin D (25-OH Vitamin D)
- Vitamin D is essential for mitochondrial function, cellular energy production, and fat metabolism. Low levels of vitamin D are often associated with insulin resistance, increased fat storage, and increased inflammation.
- Coenzyme Q10 (CoQ10)
- Essential for mitochondrial ATP production. Low levels of CoQ10 are associated with fatigue, impaired fat oxidation, and reduced mitochondrial efficiency.
- Magnesium (Serum or RBC)
- Magnesium is involved in ATP production and muscle contraction, and low levels can impair mitochondrial function and fat metabolism.
- Carnitine Levels
- Carnitine is necessary for transporting fatty acids into the mitochondria for oxidation. Low carnitine levels can hinder fat burning and mitochondrial efficiency.
7. Genetic Testing
Genetics play a role in mitochondrial function, fat metabolism, and aging. Understanding specific genetic variants can help tailor a more personalized program for mitochondrial health.
- DNA Analysis (e.g., 23andMe, Ancestry.com with genetic health reports)
- Specific SNPs (single nucleotide polymorphisms) related to mitochondrial function, fat metabolism (like PPAR-alpha, UCP1), and longevity (like sirtuins, FOXO3) can provide insights into an individual’s predisposition for metabolic efficiency, aging, and fat loss.
- Genetic Reports on Mitochondrial Health
- Certain genes (e.g., SIRT1, AMPK, UCP2) are involved in mitochondrial biogenesis and fat oxidation. Testing for these genes can help assess how well your body is able to handle metabolic stress, mitochondrial health, and fat loss.
Conclusion
By combining these functional lab tests, you can create a more personalized and holistic approach to mitochondrial health, fat loss, and longevity optimization. Each test can provide insights into mitochondrial function, nutritional status, hormonal balance, insulin sensitivity, and gut health, allowing for a tailored program to support metabolic flexibility, optimal fat burning, and long-term health.
To oxidize fat effectively and enhance the process of fat metabolism during exercise, several strategies can be employed:
How to Oxidize Fat
- Aerobic Exercise: Engaging in low to moderate-intensity aerobic exercises (like walking, jogging, or cycling) encourages the body to utilize fat as a primary fuel source. These activities should be sustained for longer durations (typically 30 minutes or more) to maximize fat oxidation.
- Longer Training Sessions: Incorporating longer training sessions helps deplete glycogen stores, which shifts the body’s metabolism toward fat oxidation.
- Proper Nutrition:
- Low-Carbohydrate Diets: Adopting a lower carbohydrate intake can enhance the body’s ability to utilize fat for energy. However, this should be approached with caution and tailored to individual needs.
- Incorporating Healthy Fats: Consuming healthy fats (like avocados, nuts, seeds, and olive oil) can help promote fat oxidation by providing a source of fuel.
- Interval Training: Including high-intensity interval training (HIIT) can improve mitochondrial density and efficiency, enhancing the body’s ability to oxidize fat over time.
- Adequate Recovery: Ensuring proper recovery between workouts allows the body to adapt and become more efficient at fat oxidation.
- Supplementation: Some supplements (like L-carnitine, MCT oil, or certain amino acids) may support fat oxidation, but it’s essential to consult a healthcare professional before starting any supplementation regimen.
Fuel Sources Used by Mitochondria to Create ATP
During exercise, the primary fuel sources used by mitochondria in muscle cells to generate ATP (adenosine triphosphate) include:
- Carbohydrates: Glucose, derived from dietary carbohydrates or glycogen stores in muscles and the liver, is a primary energy source, particularly during high-intensity exercise.
- Fatty Acids: Fat stores (triglycerides) are broken down into free fatty acids, which can be oxidized in the mitochondria to produce ATP. This process is especially significant during prolonged, moderate-intensity activities.
- Proteins: While not a primary fuel source, amino acids from protein can also be used for energy during prolonged exercise or when carbohydrate stores are low. This occurs through a process called gluconeogenesis.
- Ketones: In states of prolonged fasting or very low carbohydrate intake (such as in ketogenic diets), ketone bodies produced from fatty acids can serve as an alternative fuel source for the brain and muscles.
In summary, promoting fat oxidation involves a combination of exercise, nutrition, and recovery strategies, while the mitochondria primarily use carbohydrates and fats to produce ATP during exercise.
Lactate plays a significant role in energy metabolism, especially during exercise. Here’s how it contributes to fuel utilization in the body:
Role of Lactate as Fuel
- Byproduct of Anaerobic Glycolysis: During high-intensity exercise, when oxygen availability is limited, glucose is metabolized through anaerobic glycolysis, leading to the production of lactate. This process helps generate ATP quickly, which is essential for sustaining high levels of effort.
- Lactate Shuttle: Lactate is not merely a waste product; it can be utilized as a fuel source. The lactate shuttle theory suggests that lactate produced in one tissue (like skeletal muscle) can be transported to other tissues (such as the heart and liver) where it can be converted back into pyruvate and used for energy production or glucose synthesis.
- Fuel for the Heart: The heart is particularly adept at using lactate as a fuel source. It can oxidize lactate to produce ATP, especially during prolonged exercise when glucose and fatty acid availability may be reduced.
- Lactate and Gluconeogenesis: Lactate can be converted back to glucose in the liver through a process called gluconeogenesis. This is particularly important during prolonged exercise or fasting, as it helps replenish glycogen stores and maintain blood glucose levels.
- Regulation of pH: Although lactate is often associated with muscle fatigue, it actually helps regulate acidity in the muscle during intense exercise by buffering hydrogen ions. This allows for sustained performance despite the accumulation of metabolic byproducts.
- Role in Recovery: After exercise, lactate can be cleared from the bloodstream and used as a fuel source during the recovery phase, aiding in the restoration of energy levels and promoting the healing of muscle tissue.
Summary
In summary, lactate serves as an important fuel source during and after exercise. It supports energy production during high-intensity efforts, can be transported to various tissues for oxidation, and contributes to the metabolic processes that sustain exercise performance and recovery. By utilizing lactate effectively, the body can improve its endurance and overall metabolic efficiency.
Optimizing Fat Oxidation and Energy Production During Exercise
As athletes and fitness enthusiasts, understanding how our bodies utilize different fuel sources during exercise can significantly enhance performance and recovery. This post will delve into the process of fat oxidation, the various fuel sources used by mitochondria to create ATP (adenosine triphosphate), and the important role of lactate as a fuel.
How to Oxidize Fat Effectively
To maximize fat oxidation and improve metabolic efficiency during exercise, consider the following strategies:
- Aerobic Exercise: Engaging in low to moderate-intensity aerobic activities—like walking, jogging, or cycling—encourages the body to utilize fat as a primary fuel source. Sustaining these activities for longer durations (typically 30 minutes or more) will help maximize fat oxidation.
- Longer Training Sessions: Incorporating longer training sessions helps deplete glycogen stores, prompting the body to shift its metabolism toward fat oxidation.
- Proper Nutrition:
- Low-Carbohydrate Diets: Adopting a lower carbohydrate intake can enhance the body’s ability to utilize fat for energy, although this should be tailored to individual needs.
- Incorporating Healthy Fats: Consuming healthy fats (like avocados, nuts, seeds, and olive oil) can support fat oxidation by providing a steady source of fuel.
- Interval Training: Including high-intensity interval training (HIIT) can improve mitochondrial density and efficiency, ultimately enhancing the body’s ability to oxidize fat over time.
- Adequate Recovery: Ensuring proper recovery between workouts allows the body to adapt and become more efficient at fat oxidation.
- Supplementation: Some supplements (like L-carnitine, MCT oil, or certain amino acids) may support fat oxidation, but it’s essential to consult a healthcare professional before starting any supplementation regimen.
Fuel Sources Used by Mitochondria to Create ATP
During exercise, mitochondria in muscle cells utilize various fuel sources to generate ATP, the energy currency of the body:
- Carbohydrates: Glucose, derived from dietary carbohydrates or glycogen stores in muscles and the liver, is a primary energy source, especially during high-intensity exercise.
- Fatty Acids: Fat stores (triglycerides) are broken down into free fatty acids, which are oxidized in the mitochondria to produce ATP. This process is particularly significant during prolonged, moderate-intensity activities.
- Proteins: While not a primary fuel source, amino acids from protein can be used for energy during prolonged exercise or when carbohydrate stores are low, primarily through gluconeogenesis.
- Ketones: In states of prolonged fasting or very low carbohydrate intake (such as in ketogenic diets), ketone bodies produced from fatty acids can serve as an alternative fuel source for the brain and muscles.
The Role of Lactate as Fuel
Lactate is often misunderstood as merely a byproduct of anaerobic metabolism. However, it plays a crucial role in energy production during exercise:
- Byproduct of Anaerobic Glycolysis: During high-intensity exercise, when oxygen availability is limited, glucose is metabolized through anaerobic glycolysis, resulting in the production of lactate. This process allows for rapid ATP generation essential for sustaining high levels of effort.
- Lactate Shuttle: Lactate produced in one tissue (like skeletal muscle) can be transported to other tissues (such as the heart and liver), where it can be converted back into pyruvate and used for energy production or glucose synthesis.
- Fuel for the Heart: The heart is particularly adept at utilizing lactate as a fuel source, oxidizing it to produce ATP, especially during prolonged exercise when glucose and fatty acid availability may decrease.
- Lactate and Gluconeogenesis: Lactate can be converted back to glucose in the liver through gluconeogenesis, helping replenish glycogen stores and maintain blood glucose levels during prolonged exercise or fasting.
- Regulation of pH: While lactate is associated with muscle fatigue, it helps regulate acidity in the muscle during intense exercise by buffering hydrogen ions, allowing for sustained performance.
- Role in Recovery: After exercise, lactate can be cleared from the bloodstream and used as a fuel source during recovery, aiding in the restoration of energy levels and promoting muscle healing.
Conclusion
Understanding how to optimize fat oxidation and effectively utilize various fuel sources, including lactate, can enhance performance and recovery in athletes. By incorporating aerobic exercise, interval training, and proper nutrition, along with recognizing the crucial role of lactate in energy metabolism, you can support your body’s ability to perform at its best. With these insights, you can train smarter, recover better, and achieve your fitness goals more efficiently.
Sparing glycogen during endurance exercise is essential for maximizing performance and delaying fatigue. Here are several strategies to help spare glycogen stores while maintaining energy levels during prolonged activities:
1. Train at the Right Intensity
- Aerobic Base Training: Focus on building an aerobic base through low to moderate-intensity training. This helps increase mitochondrial density and improves the body’s ability to utilize fat as a fuel source, reducing reliance on glycogen.
- Use Zone Training: Implement heart rate zone training to ensure that you are training primarily in the fat-burning zone (typically 60-70% of maximum heart rate). This promotes fat oxidation and spares glycogen.
2. Increase Fat Utilization
- Fat Adaptation: Incorporate training sessions that encourage fat oxidation. This can be achieved through a low-carbohydrate, high-fat (LCHF) diet or by including longer, slower training sessions where the body is encouraged to use fat for fuel.
- MCT Oil and Exogenous Ketones: Using medium-chain triglycerides (MCTs) or exogenous ketones can provide a quick source of fat-derived energy without significantly impacting glycogen stores.
3. Carbohydrate Loading
- Pre-Exercise Glycogen Loading: Prior to endurance events, engage in carbohydrate loading (increasing carbohydrate intake a few days before an event) to maximize glycogen stores. This can delay fatigue during prolonged exercise.
- Incorporate Carbohydrates Strategically: During training and races, consume carbohydrates strategically rather than continuously. This can include consuming a small amount before hitting glycogen-depleting zones.
4. Utilize Lactate as Fuel
- Train to Increase Lactate Threshold: Focus on increasing your lactate threshold through interval training or tempo runs. Higher lactate thresholds allow your body to utilize lactate more effectively, which can spare glycogen.
5. Optimal Hydration and Electrolytes
- Stay Hydrated: Adequate hydration can help maintain blood flow and improve the efficiency of energy utilization. Dehydration can lead to increased reliance on glycogen stores.
- Electrolyte Balance: Maintain electrolyte balance, particularly sodium and potassium, to support overall performance and energy production.
6. Pre-Exercise Nutrition
- Balanced Pre-Workout Meal: Consume a balanced meal or snack before exercise that includes carbohydrates, healthy fats, and protein. This provides readily available energy and can help spare glycogen during workouts.
- Timing: Aim to eat 2-3 hours before a workout to optimize digestion and energy availability.
7. Post-Exercise Recovery
- Post-Workout Nutrition: Focus on replenishing glycogen stores with a combination of carbohydrates and protein after exercise. This helps recover glycogen levels for future workouts.
- Adequate Recovery Time: Allow sufficient time between workouts for muscle recovery and glycogen replenishment. This ensures you start your next workout with adequate energy stores.
8. Utilize Active Recovery Techniques
- Incorporate Active Recovery: Engage in low-intensity activities post-exercise to promote blood flow and nutrient delivery, aiding in glycogen replenishment without depleting stores further.
Conclusion
By implementing these strategies, you can effectively spare glycogen stores during endurance exercise. The key lies in optimizing your training intensity, enhancing fat utilization, and strategically managing your nutrition before, during, and after workouts. This approach not only enhances performance but also allows for more sustainable energy levels throughout your endurance activities.
Fat oxidation is the process by which the body breaks down fat molecules to produce energy, specifically in the form of ATP (adenosine triphosphate), which fuels cellular functions. This process is particularly significant during prolonged, low to moderate-intensity exercise, as the body shifts toward using fat as a primary energy source instead of carbohydrates.
What is Fat Oxidation?
- Breakdown of Fat: Fat oxidation begins with the breakdown of triglycerides (fat stores) into free fatty acids and glycerol, a process known as lipolysis. These free fatty acids are then transported to cells for energy production.
- Transport into Mitochondria: Once inside the cells, free fatty acids are transported into the mitochondria—the cell’s energy powerhouses—where they undergo beta-oxidation, a metabolic process that breaks them down into smaller molecules.
- Beta-Oxidation and ATP Production: During beta-oxidation, fatty acids are broken down into acetyl-CoA molecules, which enter the citric acid cycle (Krebs cycle). This cycle generates electrons that feed into the electron transport chain, ultimately producing ATP for muscle contraction and other cellular functions.
- Sparing Glycogen: Fat oxidation is advantageous during endurance activities because it helps conserve glycogen (stored carbohydrates) for higher-intensity efforts or for when carbohydrate availability is low.
Mitochondria Function in Exercise
Mitochondria play a central role in energy production, especially during sustained, aerobic exercise. Here’s how they contribute:
- ATP Production: Mitochondria are responsible for producing ATP, which powers muscle contractions and other cellular activities. They do this through a process known as oxidative phosphorylation, where energy derived from nutrients (fats and carbohydrates) is converted into ATP.
- Aerobic Metabolism: Mitochondria are crucial for aerobic (oxygen-dependent) metabolism. During moderate-intensity exercise, they enable the body to use fat and carbohydrate stores efficiently to create energy without depleting glycogen as quickly.
- Fat Oxidation and Glycogen Sparing: Increased mitochondrial density and efficiency—often achieved through endurance training—improve the body’s ability to oxidize fat. This means that more fat can be used as fuel, sparing glycogen for when the body needs quick energy during intense bursts.
- Lactate Processing: Mitochondria also help manage lactate produced during high-intensity exercise by converting it into pyruvate, which can be used to produce more ATP or reconverted into glucose in the liver.
- Adaptation and Endurance: Endurance training enhances mitochondrial function, leading to greater mitochondrial density (more mitochondria in muscle cells), which boosts the capacity for sustained energy production. This adaptation helps improve endurance, delay fatigue, and increase overall exercise efficiency.
Summary
In essence, fat oxidation is the process of breaking down fat to produce ATP, which is maximized during lower-intensity exercise due to the aerobic capabilities of the mitochondria. Mitochondria are vital for producing ATP during exercise, especially for endurance athletes, as they support fat oxidation, lactate processing, and energy efficiency. With enhanced mitochondrial function, athletes experience better endurance, reduced fatigue, and a more efficient use of stored energy.
Oxidative Phosphorylation and Fat Metabolism
Oxidative phosphorylation is the final stage of cellular respiration in which mitochondria produce ATP, our body’s primary energy molecule, from nutrients like fats and carbohydrates. Here’s how this complex process works:
- Electron Transport Chain (ETC): Within the inner membrane of mitochondria, the ETC consists of protein complexes that transfer electrons derived from nutrients. Electrons are donated by NADH and FADH₂ (produced from earlier metabolic steps, including beta-oxidation of fats), which move through the ETC, releasing energy.
- Proton Gradient: The energy from electrons is used to pump protons (H⁺ ions) from the mitochondrial matrix into the intermembrane space, creating a high concentration of protons outside the inner membrane. This setup generates an electrochemical gradient.
- ATP Synthase: Protons flow back into the matrix through the enzyme ATP synthase, which uses the energy from this movement to combine ADP (adenosine diphosphate) and inorganic phosphate (Pi) to form ATP.
- Oxygen as Final Electron Acceptor: Oxygen is essential here because it acts as the final electron acceptor at the end of the ETC, combining with electrons and protons to form water. This is why oxygen availability is critical for efficient ATP production and aerobic metabolism.
Fat Metabolism and Oxidative Phosphorylation
Fat metabolism in the mitochondria involves converting stored fat into ATP primarily through oxidative phosphorylation, especially during prolonged, low to moderate-intensity activities. Here’s how:
- Beta-Oxidation: Fatty acids from stored triglycerides undergo beta-oxidation, which breaks down long fatty acid chains into acetyl-CoA molecules in the mitochondrial matrix.
- Acetyl-CoA in the Citric Acid Cycle: The acetyl-CoA enters the citric acid cycle (Krebs cycle), where it generates electron carriers (NADH and FADH₂) that fuel oxidative phosphorylation.
- ATP Generation: The electron carriers produced are then used in oxidative phosphorylation to generate ATP, providing a steady, long-lasting source of energy, primarily at lower exercise intensities when the body prefers fat as fuel.
Carbohydrate Metabolism Activation
Carbohydrate metabolism becomes increasingly important as exercise intensity rises. Here’s why and when it’s activated:
- Quick Energy from Glycolysis: Carbohydrate metabolism starts with glycolysis, which breaks down glucose or stored glycogen into pyruvate, producing small amounts of ATP quickly. This process can operate with or without oxygen, making it useful for both aerobic and anaerobic exercise.
- High-Intensity Exercise: As exercise intensity increases (above 65-70% of VO₂ max), the demand for ATP outpaces the rate at which fats can be oxidized, so the body starts relying more on carbohydrates. Carbohydrates can be metabolized faster than fats, which is why they are preferred at higher intensities.
- Lactate Production in Anaerobic Glycolysis: During very high-intensity efforts, oxygen becomes limited, and pyruvate from glycolysis is converted to lactate instead of entering the mitochondria. This allows glycolysis to continue producing ATP quickly, but lactate buildup can contribute to muscle fatigue over time.
- Carbohydrate Storage and Glycogen Depletion: Since glycogen is limited (stored in muscles and the liver), carbohydrate metabolism is finite. Sparing glycogen is critical during endurance events to avoid fatigue, which is why trained athletes try to improve their fat oxidation capabilities for longer-lasting energy.
Summary
- Oxidative Phosphorylation is the primary ATP-generating process in the mitochondria, especially during aerobic exercise.
- Fat Metabolism is efficient at lower intensities, producing sustained energy through oxidative phosphorylation.
- Carbohydrate Metabolism is activated at higher intensities, as it provides quick energy via glycolysis and can produce ATP both aerobically and anaerobically.
Glycolysis occurs in the cytoplasm (or cytosol) of muscle cells. This is the fluid portion of the cell outside of the mitochondria where various metabolic reactions take place.
Here’s how glycolysis fits into carbohydrate metabolism in muscles:
- Breakdown of Glucose and Glycogen: Carbohydrate metabolism starts with glycolysis, where glucose (from blood glucose or broken down from stored glycogen) is split into two molecules of pyruvate through a series of enzymatic steps.
- ATP Production: Glycolysis generates a small amount of ATP quickly without needing oxygen, making it ideal for both aerobic and anaerobic activities. Each molecule of glucose produces a net gain of 2 ATP molecules during glycolysis.
- Pyruvate Pathways:
- Aerobic Conditions: If oxygen is available (typical of lower-intensity exercise), pyruvate is transported into the mitochondria, where it enters the citric acid cycle and oxidative phosphorylation to produce more ATP.
- Anaerobic Conditions: During high-intensity exercise when oxygen is limited, pyruvate is converted to lactate in the cytoplasm. This process allows glycolysis to continue generating ATP rapidly, although lactate buildup can contribute to muscle fatigue.
By starting in the cytoplasm, glycolysis provides a quick source of energy to the muscle cells, which can then be used directly for muscle contractions or feed into aerobic pathways in the mitochondria for sustained energy.
mitochondria are not solely responsible for fat oxidation; they also play a crucial role in carbohydrate oxidation and, to a lesser extent, protein metabolism to produce ATP. The mitochondria’s main function is to carry out oxidative phosphorylation, a process that converts energy from various fuel sources (not just fats) into ATP.
Fuel Sources for ATP Production in Mitochondria
- Fat Oxidation:
- Beta-Oxidation: Mitochondria oxidize fatty acids through beta-oxidation, which breaks down fats into acetyl-CoA, feeding into the citric acid cycle and ultimately producing ATP.
- This is most active during low to moderate-intensity exercise, where fat can serve as a primary energy source for ATP.
- Carbohydrate Oxidation:
- Pyruvate Oxidation: Glycolysis in the cytoplasm breaks down glucose to pyruvate, which then enters the mitochondria if oxygen is available.
- Citric Acid Cycle: Pyruvate is converted into acetyl-CoA, which enters the citric acid cycle, generating electron carriers (NADH and FADH₂) that fuel the electron transport chain for ATP production.
- Carbohydrate oxidation is faster than fat oxidation, so it becomes the dominant ATP source during moderate to high-intensity exercise.
- Protein Oxidation (less common):
- Amino Acids: When glycogen and fat stores are low, certain amino acids can enter the citric acid cycle as intermediates. However, this is generally a last-resort energy source and is not as efficient as fat or carbohydrate oxidation.
- Protein oxidation is typically minimal but may increase during prolonged endurance exercise or starvation.
Summary
Mitochondria are versatile energy producers, using fats, carbohydrates, and occasionally proteins to generate ATP. The fuel source used depends on factors like exercise intensity, duration, and energy availability.
The amount of ATP produced varies depending on the fuel source and the pathway used. Here’s a breakdown:
1. ATP Production from Carbohydrate (Glucose) Oxidation
- Glycolysis (in the cytoplasm):
- Net ATP: 2 ATP per glucose molecule
- Produces 2 NADH (converted later to ATP in mitochondria)
- Pyruvate Oxidation and Citric Acid Cycle (in mitochondria):
- Each glucose yields 2 pyruvate molecules, which produce:
- 2 ATP (directly from the citric acid cycle)
- 8 NADH (6 from the citric acid cycle, 2 from pyruvate oxidation)
- 2 FADH₂
- Each glucose yields 2 pyruvate molecules, which produce:
- Electron Transport Chain (ETC) / Oxidative Phosphorylation:
- Each NADH = ~2.5 ATP → 10 NADH x 2.5 = 25 ATP
- Each FADH₂ = ~1.5 ATP → 2 FADH₂ x 1.5 = 3 ATP
- Total ATP from One Glucose Molecule:
- Approximately 30-32 ATP (including all pathways)
2. ATP Production from Fatty Acid (Palmitate) Oxidation
- Beta-Oxidation of Palmitate (16-carbon fatty acid):
- Palmitate undergoes 7 cycles of beta-oxidation, yielding:
- 8 Acetyl-CoA (enters citric acid cycle)
- 7 NADH and 7 FADH₂ from beta-oxidation
- Palmitate undergoes 7 cycles of beta-oxidation, yielding:
- Citric Acid Cycle (for 8 Acetyl-CoA):
- Produces 24 NADH, 8 FADH₂, and 8 ATP
- Electron Transport Chain / Oxidative Phosphorylation:
- 31 NADH x 2.5 = 77.5 ATP
- 15 FADH₂ x 1.5 = 22.5 ATP
- Total ATP from One Palmitate Molecule:
- Approximately 106 ATP
3. ATP Production from Amino Acid Oxidation
- Varies by Amino Acid:
- Each amino acid enters the citric acid cycle at different points, so ATP yield varies widely.
- Typically ranges from 10 to 30 ATP per amino acid, but protein is generally a minor fuel source except in specific circumstances like prolonged starvation or extreme endurance exercise.
Summary Table
Fuel Source | Approximate Total ATP Yield |
---|---|
Glucose (Carbohydrate) | 30-32 ATP |
Palmitate (Fatty Acid) | 106 ATP |
Amino Acid (Varies) | 10-30 ATP (depends on amino acid) |
These numbers represent theoretical maximums under ideal conditions, and real-world ATP yields can vary.
To produce ATP effectively, the body relies on various nutrients, enzymes, and cofactors that play essential roles in metabolic pathways. Here’s a breakdown of key components:
1. Nutrients for ATP Production
- Carbohydrates: Provide glucose for glycolysis and the citric acid cycle.
- Fats: Provide fatty acids for beta-oxidation in the mitochondria.
- Proteins: Provide amino acids, which can enter the citric acid cycle under certain conditions.
- Oxygen: Essential as the final electron acceptor in the electron transport chain, allowing for oxidative phosphorylation and efficient ATP production.
2. Enzymes in ATP Production
- Glycolytic Enzymes:
- Hexokinase: Catalyzes the phosphorylation of glucose in glycolysis.
- Phosphofructokinase (PFK): A key regulatory enzyme in glycolysis, helping convert fructose-6-phosphate to fructose-1,6-bisphosphate.
- Pyruvate Kinase: Catalyzes the final step of glycolysis, forming pyruvate and ATP.
- Beta-Oxidation Enzymes:
- Acyl-CoA Dehydrogenase: Initiates the first step of fatty acid oxidation.
- Enoyl-CoA Hydratase and Beta-Hydroxyacyl-CoA Dehydrogenase: Aid in further steps of beta-oxidation.
- Citric Acid Cycle Enzymes:
- Citrate Synthase: Catalyzes the first step of the citric acid cycle, forming citrate from oxaloacetate and acetyl-CoA.
- Isocitrate Dehydrogenase and Alpha-Ketoglutarate Dehydrogenase: Key enzymes that produce NADH and CO₂ in the citric acid cycle.
- Electron Transport Chain (ETC) Enzymes:
- NADH Dehydrogenase (Complex I): Transfers electrons from NADH to the ETC.
- Cytochrome c Oxidase (Complex IV): Catalyzes the final step of electron transfer to oxygen.
3. Essential Cofactors and Coenzymes
- NAD+ (Nicotinamide Adenine Dinucleotide) and FAD (Flavin Adenine Dinucleotide):
- Act as electron carriers, receiving electrons in glycolysis, beta-oxidation, and the citric acid cycle, then transferring them to the ETC.
- Coenzyme A (CoA):
- Important for forming acetyl-CoA, which enters the citric acid cycle.
- ATP Synthase Co-factors:
- Magnesium (Mg²⁺): Necessary for the stability and activity of ATP synthase, the enzyme responsible for ATP production in the ETC.
4. Vitamins and Minerals Supporting ATP Production
- B Vitamins:
- B1 (Thiamine): Vital for pyruvate dehydrogenase, which converts pyruvate to acetyl-CoA.
- B2 (Riboflavin): Required for FAD and FADH₂ production.
- B3 (Niacin): Used to produce NAD⁺ and NADH, important electron carriers.
- B5 (Pantothenic Acid): Essential for coenzyme A synthesis.
- B6 (Pyridoxine): Involved in amino acid metabolism and supports gluconeogenesis.
- Magnesium (Mg²⁺): Required for ATP binding and activation in enzymatic reactions.
- Iron (Fe): A component of the cytochromes in the electron transport chain.
- Copper (Cu): Essential for certain enzymes in the electron transport chain.
- Phosphorus: Integral to ATP structure (adenosine triphosphate).
5. Additional Supportive Compounds
- L-Carnitine: Assists in transporting fatty acids into the mitochondria for beta-oxidation.
- Creatine Phosphate: Quickly regenerates ATP from ADP in muscle cells during short, high-intensity exercise.
- Coenzyme Q10 (Ubiquinone): Acts within the electron transport chain to transfer electrons between complexes I and III.
Summary
Efficient ATP production requires a complex synergy of nutrients (carbohydrates, fats, proteins, and oxygen), enzymes, cofactors like NAD⁺ and FAD, vitamins (especially B vitamins), and minerals such as magnesium and iron. Each component plays a critical role in maintaining energy balance and supporting metabolic health during all activities, especially exercise.
AMPK Activation, Mitochondrial Function, and Fat Oxidation with Avere-TRIM Ingredients
AMPK (AMP-activated protein kinase) is a cellular energy sensor and metabolic regulator that plays a crucial role in maintaining energy balance. It enhances mitochondrial biogenesis, fatty acid oxidation, and glucose uptake while inhibiting fat storage and inflammation. Many natural compounds can stimulate AMPK, leading to improved metabolic efficiency, fat oxidation, and mitochondrial function at rest and during exercise.
How AMPK Enhances Fat Oxidation & Mitochondrial Function
- Fat Oxidation: AMPK activation increases fatty acid uptake into the mitochondria by upregulating CPT1 (carnitine palmitoyltransferase 1), which enhances fat oxidation.
- Mitochondrial Biogenesis: AMPK enhances PGC-1α (peroxisome proliferator-activated receptor gamma coactivator 1-alpha), which stimulates the formation of new mitochondria, improving endurance and energy production.
- Glucose Metabolism: AMPK enhances glucose uptake by increasing GLUT4 translocation, improving insulin sensitivity and energy availability.
- Anti-Inflammatory & Longevity Benefits: By reducing oxidative stress and inflammation, AMPK activation supports overall cellular health and longevity.
Key Phytonutrients in Avere-TRIM and Their Role in AMPK Activation
- Quercetin
- Stimulates AMPK activation and mitochondrial biogenesis.
- Enhances fat oxidation by increasing SIRT1 and PGC-1α activity.
- Improves endurance by reducing oxidative stress and enhancing oxygen utilization.
- Curcumin
- Activates AMPK and promotes mitochondrial function.
- Reduces inflammation and oxidative stress, which can impair metabolic efficiency.
- Enhances fat oxidation by increasing fatty acid mobilization and utilization.
- Berberine
- One of the most potent AMPK activators, often compared to Metformin.
- Increases glucose uptake, reduces insulin resistance, and enhances mitochondrial function.
- Boosts fatty acid oxidation and inhibits fat storage.
- Resveratrol
- Stimulates AMPK and SIRT1, supporting mitochondrial biogenesis and fat metabolism.
- Increases endurance and metabolic flexibility by enhancing energy production.
- Protects against oxidative stress and supports cardiovascular health.
- Green Tea Extract (EGCG)
- Increases AMPK activation and enhances mitochondrial function.
- Stimulates thermogenesis and fat oxidation by upregulating norepinephrine activity.
- Supports metabolic efficiency during exercise and at rest.
- L-Theanine
- Modulates AMPK activation indirectly by reducing stress-related cortisol spikes.
- Supports mitochondrial health by reducing oxidative stress and improving cellular resilience.
- Enhances cognitive function and focus, which may aid in exercise performance.
- Moringa Leaf
- Stimulates AMPK and enhances glucose metabolism.
- Provides polyphenols and antioxidants that support mitochondrial function.
- Supports anti-inflammatory pathways that enhance metabolic health.
Liposomal Delivery & Phosphatidylcholine for Absorption
Avere-TRIM uses a liposomal delivery system with Non-GMO European sunflower phosphatidylcholine to enhance bioavailability and cellular uptake. Phosphatidylcholine supports mitochondrial membrane integrity, optimizing energy production and fat metabolism.
Summary of Benefits for AMPK Activation & Mitochondrial Function
Ingredient | AMPK Activation | Mitochondrial Support | Fat Oxidation |
---|---|---|---|
Quercetin | ✅ | ✅ | ✅ |
Curcumin | ✅ | ✅ | ✅ |
Berberine | ✅✅✅ | ✅✅ | ✅✅✅ |
Resveratrol | ✅✅ | ✅✅✅ | ✅✅ |
Green Tea (EGCG) | ✅✅ | ✅ | ✅✅ |
L-Theanine | ✅ | ✅ | ✅ |
Moringa Leaf | ✅ | ✅ | ✅ |
Effects at Rest vs. During Exercise
- At Rest: AMPK activation increases basal metabolic rate, improves insulin sensitivity, and enhances mitochondrial efficiency.
- During Exercise: Fat oxidation is enhanced, mitochondrial ATP production is optimized, and endurance capacity is improved.
Conclusion
Avere-TRIM’s formulation is strategically designed to activate AMPK, enhance mitochondrial function, and optimize fat oxidation. This is beneficial for metabolic health, endurance, and body recomposition—supporting both performance and longevity. The liposomal delivery system further ensures maximum absorption of these powerful compounds.
Determining the optimal dosages of specific phytonutrients to activate AMPK, enhance mitochondrial function, and promote fat oxidation involves analyzing available research. Below is a summary of findings for each compound:
1. Quercetin
- Dosage: Studies have utilized doses ranging from 500 mg to 1,000 mg per day. Doses up to 1 gram daily have been used safely for up to 12 weeks.
2. Curcumin
- Dosage: Effective doses in studies vary, but commonly range from 500 mg to 2,000 mg per day. Curcumin’s bioavailability is low; thus, formulations with enhanced absorption or co-administration with piperine are often used.
3. Berberine
- Dosage: Commonly studied doses are 500 mg taken two to three times daily, totaling 1,000 mg to 1,500 mg per day. This regimen has been shown to activate AMPK and improve metabolic parameters.
4. Resveratrol
- Dosage: Research indicates that doses between 150 mg to 500 mg per day may be effective. Higher doses are sometimes used, but long-term safety data is limited.
5. Green Tea Extract (EGCG)
- Dosage: Effective doses of EGCG range from 400 mg to 800 mg per day. Green tea extracts are generally considered safe even at higher doses, but excessive intake may lead to adverse effects.
6. L-Theanine
- Dosage: Typical doses range from 100 mg to 400 mg per day. L-Theanine is generally well-tolerated and considered safe within this range.
7. Moringa Leaf
- Dosage: Research on moringa leaf is less specific regarding standardized dosing. However, studies have used doses ranging from 500 mg to 2,000 mg per day, depending on the extract’s concentration and the study’s focus.
Considerations:
- Bioavailability: Many of these compounds have low natural bioavailability. Utilizing liposomal delivery systems, as mentioned in your formulation, can enhance absorption.
- Safety: While these compounds are generally considered safe, it’s essential to monitor for potential side effects, especially at higher doses. Consulting with a healthcare professional before starting any new supplementation regimen is advisable.
In summary, the dosages mentioned above are based on current research and have been associated with activating AMPK, enhancing mitochondrial function, and promoting fat oxidation. Individual responses may vary, and it’s crucial to consider factors such as bioavailability, potential interactions, and overall health status when determining the appropriate dosage.
AMPK Activation, Mitochondrial Function, and Fat Oxidation with Avere-TRIM Ingredients
AMPK (AMP-activated protein kinase) is a cellular energy sensor and metabolic regulator that plays a crucial role in maintaining energy balance. It enhances mitochondrial biogenesis, fatty acid oxidation, and glucose uptake while inhibiting fat storage and inflammation. Many natural compounds can stimulate AMPK, leading to improved metabolic efficiency, fat oxidation, and mitochondrial function at rest and during exercise.
How AMPK Enhances Fat Oxidation & Mitochondrial Function
- Fat Oxidation: AMPK activation increases fatty acid uptake into the mitochondria by upregulating CPT1 (carnitine palmitoyltransferase 1), which enhances fat oxidation.
- Mitochondrial Biogenesis: AMPK enhances PGC-1α (peroxisome proliferator-activated receptor gamma coactivator 1-alpha), which stimulates the formation of new mitochondria, improving endurance and energy production.
- Glucose Metabolism: AMPK enhances glucose uptake by increasing GLUT4 translocation, improving insulin sensitivity and energy availability.
- Anti-Inflammatory & Longevity Benefits: By reducing oxidative stress and inflammation, AMPK activation supports overall cellular health and longevity.
Effects at Rest vs. During Exercise
- At Rest: AMPK activation increases basal metabolic rate, improves insulin sensitivity, and enhances mitochondrial efficiency.
- During Exercise: Fat oxidation is enhanced, mitochondrial ATP production is optimized, and endurance capacity is improved.
Conclusion
Avere-TRIM’s formulation is strategically designed to activate AMPK, enhance mitochondrial function, and optimize fat oxidation. This is beneficial for metabolic health, endurance, and body recomposition—supporting both performance and longevity. The liposomal delivery system further ensures maximum absorption of these powerful compounds.
Action Plan: Optimize Cellular Health for Longevity, Fat Metabolism & Performance
To improve body composition, metabolic efficiency, and longevity at the cellular level, focus on mitochondrial function, gut health, inflammation control, and metabolic flexibility. Here’s how to get started:
1️⃣ Mitochondrial Optimization for Energy & Fat Metabolism
✅ Increase Mitochondrial Biogenesis & Efficiency
- Zone 2 aerobic training (low-intensity steady-state) 3–5x/week to maximize fat oxidation.
- HIIT & sprint intervals 1–2x/week to stimulate mitochondrial density.
- Cold exposure (55–57°F for 2–5 min, 3–5x/week) to activate mitochondrial biogenesis via PGC-1α.
- Heat exposure (sauna 2–4x/week, 15–20 min at 170°F) to increase heat shock proteins and mitochondrial resilience.
- Red light therapy & sun exposure for mitochondrial activation and ATP production.
✅ Boost Fat Oxidation & Metabolic Flexibility
- Start the day with fasted low-intensity movement (walk, yoga, or Zone 2 training) to enhance fat adaptation.
- Use targeted carbohydrate intake for workouts (30g pre-workout for endurance, 15g protein pre-strength training).
- Include mitochondrial-supporting nutrients: CoQ10, PQQ, alpha-lipoic acid, magnesium, and creatine.
✅ Reduce Oxidative Stress & Improve Recovery
- Prioritize sleep (7–9 hours) – mitochondrial repair occurs during deep sleep.
- Use adaptogens (Rhodiola, Ashwagandha, Cordyceps) to reduce stress load.
- Eat colorful, polyphenol-rich foods (berries, dark chocolate, green tea) to combat oxidative stress.
2️⃣ Gut Health & Inflammation Control (Affects Mitochondria & Longevity)
✅ Support a Healthy Gut Microbiome for Fat Metabolism & Energy
- Increase fermentable fiber intake (resistant starch, green bananas, cooked & cooled potatoes, oats, artichokes, flaxseeds).
- Prebiotic & probiotic-rich foods (kimchi, sauerkraut, yogurt, kefir, miso) for microbiome balance.
- Consider butyrate supplementation or SCFA-enhancing probiotics (Akkermansia, Clostridium butyricum) if gut dysbiosis is present.
✅ Reduce Chronic Inflammation (Linked to Mitochondrial Dysfunction & Fat Storage)
- Test & monitor inflammation markers (hs-CRP, IL-6, Zonulin, LPS, oxidative stress markers).
- Remove inflammatory triggers: processed foods, industrial seed oils, excessive sugar.
- Increase omega-3 intake (wild-caught salmon, sardines, fish oil supplements) to counter inflammation.
- Optimize gut barrier integrity with L-glutamine, bone broth, colostrum, and zinc carnosine.
3️⃣ Hormonal & Metabolic Health for Longevity & Performance
✅ Enhance Insulin Sensitivity & Metabolic Flexibility
- Strength train 3–4x/week to improve glucose uptake and fat utilization.
- Prioritize protein intake (1.6–2.2g/kg body weight) for muscle retention and metabolic health.
- Use time-restricted eating (TRE, 12–14 hrs fasting) if beneficial for metabolic flexibility.
✅ Support Women’s Hormonal Health (Stacy Sims Approach)
- Women in perimenopause & menopause:
- Increase protein (40–60g post-workout) to combat anabolic resistance.
- Prioritize creatine & leucine-rich foods for muscle & mitochondrial support.
- Adjust fueling strategies: Avoid prolonged fasting, focus on post-workout recovery carbs & protein.
4️⃣ Key Functional Lab Tests to Personalize Your Plan
✅ Mitochondrial & Metabolic Health Tests:
- DUTCH Test → Cortisol & sex hormone balance
- Organic Acids Test (OAT) → Mitochondrial efficiency & nutrient deficiencies
- VO2 Max & FatMax Test → Find your peak fat oxidation zone for training
✅ Gut Health & Inflammation Tests:
- GI-MAP → Dysbiosis, leaky gut, inflammation
- Zonulin & LPS → Gut permeability & endotoxin load
- hs-CRP & IL-6 → Systemic inflammation markers
✅ Genetic Testing for Longevity & Fat Metabolism:
- APOE (Lipid metabolism & brain health)
- PPARGC1A (Mitochondrial biogenesis efficiency)
- FTO (Fat metabolism & insulin resistance risk)
5️⃣ Lifestyle Strategies for Longevity & Fat Loss
✅ Optimize Sleep & Circadian Rhythm
- Morning sunlight exposure (10–20 min) to regulate melatonin & mitochondria.
- Consistent sleep-wake schedule to enhance recovery & hormonal balance.
- Minimize blue light at night (wear blue light-blocking glasses, reduce screens).
✅ Manage Stress & Nervous System Regulation
- Daily breathwork, meditation, or HRV training to lower cortisol.
- Nature exposure & grounding (barefoot walking) to support mitochondrial function.
✅ Hydration & Mineral Support for Cellular Energy
- Electrolyte balance (sodium, magnesium, potassium) for mitochondrial efficiency.
- Avoid excessive alcohol & caffeine which impair mitochondrial function.
Summary: How to Get Started Today
✅ Move daily (Zone 2, strength training, HIIT) to build mitochondrial density.
✅ Fuel smartly with protein, healthy fats, and strategic carbs for training.
✅ Reduce inflammation through gut health, nutrient-dense foods, and lifestyle changes.
✅ Optimize sleep, stress, and recovery for long-term metabolic health.
✅ Get personalized functional lab testing to fine-tune your plan.
Take action now to age stronger, leaner, and more resilient!